Nitrogen fertiliser just where does it all go?
Background
Whilst soil organic matter (SOM) and crop residues are important sources of nitrogen (N) it is widely recognised that this source alone is not sufficient in itself for productive cropping without supplementation from inorganic or organic fertilisers. Nutrient management, especially N, underpins the productivity and profitability of all Australian grain production systems with fertiliser inputs comprising one of the single largest variable input costs for many growers. One tonne of N currently costs in Australia the same as 2.5 - 3 tonnes of harvested grain. Despite its critical importance for on-farm profitability, growers, advisers and researchers alike across Australia still struggle with making ‘the right decision’ about N fertiliser management.The end game is to increase nitrogen use efficiency (NUE) i.e. the efficiency with which soil mineral N is converted into grain N. The mineral N comes from fertiliser, crop residues, manures, and soil organic matter (SOM), but it is the efficiency of conversion of fertiliser into grain that is generally of greatest concern to growers. Efficiency is reduced by seasonal conditions, crop diseases, losses of N from the soil as gases, N leaching or immobilisation of N into organic forms.
Immobilisation
Immobilisation of N may occur when plant residues of low N content (e.g. wheat or sorghum straw) are decomposing in the soil. Immobilisation represents a temporary unavailability of mineral N in the soil for growing plants to access. Applying fertiliser N in a band separated from crop residues is one means of reducing immobilisation.Leaching
Leaching is the downward movement of nitrate (NO3-) with water in the soil. Loss of soil N from the plant root zone is more likely in coarse textured (sandy) soils than clay soils. Nitrate is a ‘mobile’ form of N, i.e. it can be transported in water through the soil profile, the ammonium (NH4+) form of N is not mobile.Denitrification
Denitrification occurs in saturated soils when nitrate (NO3-) is converted by soil microorganisms into the gases nitric oxide (NO), nitrous oxide (N2O) and di-nitrogen (N2). The loss of mineral N from the soil varies according to conditions and whether there is also a ready source of labile (or readily decomposable) carbon available. Di-nitrogen (N2) is the main form of the gas that is lost, but the proportion of the different gases produced is dependent on soil pH and water content.Denitrification increases as the water content (or water filled pore space – WFPS) increases above field capacity and as oxygen is consumed in the pores. Denitrification occurs more commonly and frequently in heavy textured soils, not coarse textured soils. In acidic soils, more is lost as N2O whereas in alkaline soils most is lost as N2. Once in gas form the N is no longer available to plants in the soil.
The timing of nitrogen fertilisers applied either at sowing, pre-sowing, in-crop or in some combination of these, e.g. some at sowing and some more in-crop as determined by the growing season effects the level of denitrification.
Early application is often more convenient and allows N to move lower into the root zone where it will be accessed later in the season and thus be used more for grain filling than for plant growth, but it is also then at a greater risk of denitrification loss should soil saturation/waterlogging occur. Applying N at planting can carry the risk of denitrification if heavy rains occur before plants begin to use the N from the fertiliser. In-crop applications of N fertiliser require adequate soil moisture on application and/or follow-up rainfall to ensure the applied N is taken up by the plant.
Application
Application methods include solid and liquid N products which are applied either directly to the soil surface, placed or injected into the soil (including anhydrous ammonia), or surface applied then covered in a separate operation. In-crop applications are either surface-spread as solids or sprayed as liquids. Surface applications by broadcasting may be at risk of ammonia (NH3) volatilisation, particularly in coarse textured calcareous soils and those with thick stubble layers that prevent the fertiliser from soil contact as it dissolves.Enhanced efficiency fertilisers (EEFs) are products now being promoted to growers to increase NUE. The EEFs being widely tested in Australia are urea-3,4-dimethylpyrazole phosphate (DMPP)-coated urea (commonly known as ENTEC®) and Green Urea NVTM. DMPP is a nitrification inhibitor which will slow the production of nitrate through the nitrification process i.e. the conversion of the ammonium form of mineral N (produced after the hydrolysis of urea) to the nitrate form. By reducing the amount of nitrate in the soil at any time, mineral N losses via denitrification or leaching may potentially be avoided or substantially reduced. Green Urea NVTM contains a urease inhibitor and slows the hydrolysis of urea to the ammonium form of N and potentially reduces N losses via volatilisation.
Results and discussion
To more easily explore the fate of N fertilisers, isotopically labelled (15N) fertilisers have been produced which allow us to track the movement of N applications over the growing season. After harvest, the amount of 15N in the soil, grain and residues is measured and whatever is not recovered is assumed to have been lost to the atmosphere either via denitrification or volatilisation, the latter not being the case in acid soils or if the N fertiliser has been covered or applied below the surface in alkaline soils. Leaching losses may also occur if the N is applied on coarse textured soils.In the semi-arid temperate Wimmera (annual rainfall 420 mm, alkaline vertosol), 15N fertiliser has been used to explore the interaction between the timing of N application to wheat i.e. 50kg/ha banded below the seed at sowing or topdressed at GS30 (first node) and three products (urea, Entec®, Green Urea NVTM). Whilst there was no significant difference in grain yield between the four treatments in both 2012 and 2013 (average 3.8t/ha), the data collected in the 2013 season confirmed the observations in 2012 that applying urea at GS 30 was the preferred strategy for maximising NUE, as was the use of Green Urea NVTM (Figure 1). In 2013, the late application of N fertiliser reduced N losses by 20 per cent (or 10kg N/ha).
Figure 1: Recovery of 15N (50kg N/ha) applied at Wimmera as either urea incorporated at sowing (50N), urea + DMPP applied at sowing (50EN), urea top dressed at GS30 (0:50) or urea + Urease Inhibitor applied at GS30 in the grain (50GU), straw, 0-10cm and 10-20cm of soil in 2013 (Source: Wallace 2016).
A similar observation was made in the high rainfall zone (HRZ) of Victoria (annual rainfall 685 mm, acidic chromosol) during the 2012 and 2013 seasons, with extremely high losses of applied N (77- 93kg N/ha) after deep banding 100kg N/ha of N at sowing compared to a delayed application (Figure 2). On average, 80 per cent of the N applied later in the season was recovered in either the crop or the soil.

The use of the EEF ENTEC® increased NUE in the semi-arid environment when N was applied at sowing, but had no impact when used with the later application in the HRZ. The trade-off between the additional cost of EEFs and productivity gains require further investigation.
At Wagga Wagga (annual rainfall 580 mm, acidic kandosol), the best recovery of applied N in a tillage x N experiment in 2012 was 77 per cent when only 25kg 15N/ha was top-dressed. There was no difference in yield between N application rates or tillage treatments (average 3.9t/ha) and no impact of tillage on total N recovery. Total N losses were substantially higher in 2013 where they averaged 54 per cent of applied N across rates with volatilisation suspected of being the major loss pathway (Li et al 2016).
Nitrous oxide
Nitrous oxide emissions are a relatively small loss of N from the soil to the atmosphere, mainly as a result of denitrification during saturation/waterlogging events. Total losses of nitrous oxide (N2O) as a proportion of fertiliser N applied are typically less than one per cent in dryland cropping systems but may be higher during prolonged rainfall events and waterlogging, particularly in subsoils which may have restricted drainage.
Nitrous oxide is harmful to the ozone layer and is a greenhouse gas which is 300 times more potent than carbon dioxide in terms of its global warming effect. Small emissions of nitrous oxide are normally indicative of the timing and scale of much larger N2 emissions e.g. for every one kg of N emitted as N2O after seven days of saturation, 52kg of N as N2 was lost (Grace et al., 2015).
At the Hart field site, in the SA Mid North region, results over the 2014 and 2015 seasons showed contradicting results between N application at seeding and GS31 in yield and N2O emissions. This was attributed to the distribution and amount of growing season rainfall, with higher losses again following periodic waterlogging. The strategy that maximised yield was not always the same that reduced N losses.
Ammonia volatilisation
Ammonia volatilisation is the loss of N as ammonia (NH3) from the soil to the atmosphere as a result of chemical reactions at the surface and environmental conditions. Unless it is being applied as anhydrous ammonia fertiliser, ammonia gas is only produced in the soil at a very high pH, which occurs as urea is converted to ammonium N, or when ammonium sulphate comes in contact with calcium carbonate in the soil. Soils with high clay content can buffer changes in pH and also have an affinity for ammonium adsorption, making them less at risk of converting to ammonia gas and being lost.
Ammonia volatilisation data from broadcasted N fertiliser on alkaline soils in northwest NSW is highly relevant to soils in southern Australia. Six different N products have been trialled in four different situations over two years (Figure 3). In three out of four trials on bare fallowed soils without lime, N loss from ammonium sulphate was about half that from urea, urea ammonium nitrate or green urea products. N losses from these products occurred gradually for 2-3 weeks after application of the fertiliser.
In two trials on bare fallowed soils with naturally-occurring lime (at the surface), N loss from ammonium sulphate far exceeded that from urea when applied to bare fallow soils. When N was applied as ammonium sulphate, 37 per cent of the N was lost to the atmosphere. Most of this was lost within the first 2-3 days after application. This is because ammonium sulphate chemically reacts with lime in the soil to form an unstable, high-pH compound that rapidly converts to ammonia gas which can be lost through volatilisation. N volatilisation losses from fertilisers applied as topdressing in-crop (to wheat) tended to be low, averaging just 5 per cent of the N applied.

Figure 3: Summary of the cumulative nitrogen loss via volatilisation in this study, grouped by paddock type and fertiliser treatment. Bars are means (+ standard error) of results from paddock experiments (number of paddocks in each group is given under bar). U, Urea; AS, ammonium sulfate; GU, Green Urea; UAN, urea ammonium nitrate. (Source:Schwenke 2014).
At two grass-based perennial pasture sites, substantially higher N volatilisation losses were measured from urea application compared to ammonium sulphate. Neither of these sites had lime present in the soil. Insufficient rain fell in the week after application to wash the urea through the plant and litter to the soil before it dissolved and began conversion to ammonium. The conversion process, known as hydrolysis, generates a localised high pH that results in more ammonia gas than ammonium ions, resulting in increased N volatilisation losses.
It is important to note that all of the trials were located on alkaline vertosols; medium to heavy clay soils. N volatilisation was therefore limited by rapid adsorption of ammonium onto the charged clay mineral surfaces, high soil pH buffering capacity, and when applied in-crop; canopy re-absorption of ammonia, and protection against wind at the surface.
How do these results compare to previous research? Little Australian data is available that has been collected in open field conditions which are critical factors in ammonia volatilisation. Turner et al. (2010) measured a 9.5 per cent N loss from urea and a 1.0 per cent loss from green urea applied to a wheat crop grown on a clay loam soil in the Wimmera region of Victoria. In another study, Turner et al. (2012) measured an average loss of 18 per cent from urea top-dressed into wheat or barley grown on a grey vertosol in the Mallee. Losses from ammonium sulphate and UAN applied at the same site did not exceed 6 per cent. At another site in the Wimmera, losses from urea totalled 5 per cent and 2 per cent from UAN.
Nitrogen budgets
There is a substantial interaction between the applied fertiliser and soil organic matter N which can store applied N for subsequent years. It is therefore important to consider the spatial distribution of residual banded soil N when soil testing (Figure 4).
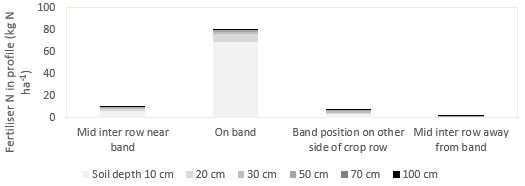
Figure 4: Spatial distribution of banded fertiliser remaining in the soil profile following harvest.
The amount of mineral N taken up by a three t/ha wheat crop with 12 per cent protein is approximately 130kg N. In southern Australia, about 40kg N/ha will slowly be supplied to the crop from stable SOM over one year. The residual effect of the previous season’s crop residues (including roots) on N supply (65-70kg N/ha for a 3t/ha wheat crop) is negated to some extent by mineralisation during the early (non-crop) months of the year and is susceptible to losses (Grace et al., 2015).
Assume that a quarter of the N mineralised from the residues is lost in the first three months (i.e. 15-20 kg N) (Grace unpublished). To close our N budget for the season we need to apply least 40-45kg N/ha before factoring in any of the loss pathways we have discussed. The 15N studies confirm that top-dressing N is the preferred option with losses of between 20-30 per cent of applied N on relatively well managed soils. The application of 50-60kg N/ha is therefore considered necessary under these circumstances. These calculations are based on a relatively ‘normal’ year. In alkaline soils, the top-dressing must be either covered or coincide with rainfall to minimise volatilisation. The use of Green Urea NVTM has been shown to reduce volatilisation without compromising yield but the additional cost of the product needs to be considered.
Conclusion
Improve your nitrogen use efficiency by:- Only applying N fertiliser when the crop needs it, not before, as large N losses can potentially occur.
- Increasing your soil organic matter levels and regularly using legumes in your rotations as both will provide a native supply of N and reduce N fertiliser requirements and increase profitability.
- Increasing your soil organic matter levels which in turn will improve your soil structure and reduce the amount of time your soils are saturated after rainfall events, and therefore, reduce the amount of time your soils are prone to large N losses.
- Regularly doing an N budget, and/or pre-sowing soil sample, to more accurately estimate your N requirements.
- For every 0.5 per cent of organic carbon (OC) (0-10cm) approximately 13kg N/ha of mineral N is supplied from soil organic matter in the top 30cm over a typical growing season (Grace et al., 2015). This N is released slowly over the growing season and not prone to losses.
- For every one tonne of cereal grain, approximately 24kg N/ha is supplied to the next crop from the crop residues. For every one tonne of legume grain, approximately 55 kg N/ha is supplied to the next crop from the residues. Most of this N is released over the first two months (when a crop is not present) and is prone to loss (Grace et al., 2015).
References
Grace, P, Armstrong, R, Harris, R, Wallace, A, Schwenke, G and Li, Guangdi, 2015Harris, R.H., Armstrong, R.D., Wallace, A.J., Belyaeva, O.N., 2016. Delaying nitrogen fertiliser application improves wheat 15N recovery from high rainfall cropping soils in south eastern Australia. Nutrient Cycling in Agroecosystems, 1-16.
Li, G.D., Conyers, M.K., Schwenke, G.D., Hayes, R.C., Liu, D.L., Lowrie, A.J., Poile, G.J., Oates, A.A., Lowrie, R.J., 2016. Tillage does not increase nitrous oxide emissions under dryland canola (Brassica napus L.) in a semiarid environment of south-eastern Australia. Soil Research 54, 512-522.
Schwenke, G.D., Manning, W., Haigh, B.M., 2014. Ammonia volatilisation from nitrogen fertilisers surface-applied to bare fallows, wheat crops and perennial-grass-based pastures on Vertosols. Soil Research 52, 805-821.
Turner D.A. (2010) Quantification and mitigation of gaseous nitrogen emissions from pasture and cropping systems. PhD Thesis, Department of Resource Management and Geography, University of Melbourne, Melbourne, Australia.
Turner, D.A., Edis, R.E., Chen, D., Freney, J.R., Denmead, O.T., 2012. Ammonia volatilization from nitrogen fertilizers applied to cereals in two cropping areas of southern Australia. Nutrient Cycling in Agroecosystems 93, 113-126.
Wallace, A.J., Armstrong, R.D., Harris, R.H., Belyaeva, O.N., Grace, P.R., Partington, D.L., (2016) In-season application of nitrogen fertiliser reduces nitrous oxide emissions from wheat grown in the medium rainfall zone of southern Australia. In press
Contact details
David RowlingsInstitute for Future Environments, Queensland University of Technology, Brisbane, Qld 4000.
07 3138 9508
d.rowlings@qut.edu.au
Was this page helpful?
YOUR FEEDBACK