NITROGEN VOLATILISATION FROM NORTHERN CROPPING SOILS
| Date: 17 Sep 2009
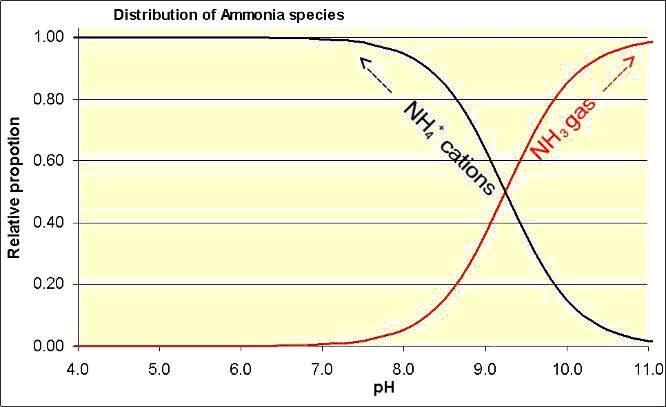
Background to the issue
In northwest NSW, ammonium sulphate (AS) is increasingly being used as an alternative to urea for nitrogen application. It has the added benefit of also supplying sulphur in a plant-available form. But, is it suitable for use as a surface applied fertiliser? Is the nitrogen subject to loss through volatilisation like urea? Volatilisation is the loss of nitrogen to the atmosphere as ammonia gas (NH3) from soils or from fertiliser applied to soil surfaces. How much is actually lost depends on (i) how much is potentially at risk of being lost, and (ii) whether the environmental conditions cause the at-risk pool to be lost. Depending on soil properties, fertiliser type and environmental conditions, losses can be as high as those of urea.
(i) Factors affecting Volatilisation Risk
(a) pH. In a soil-water solution, dissolved ammonia gas (NH3) is found in a chemical equilibrium with cations of ammonium (NH4+).
The ratio of NH4+ to NH3 in this equilibrium is highly pH dependent (see Figure 1). At low pH (more acidic) the NH4+ form dominates, while at high pH (highly alkaline) there is more of the NH3 gas form. The proportions of NH3 and NH4+ are equal at a pH of 9.2.
Figure 1. Distribution of ammonium / ammonia according to soil solution pH.
(b) Temperature. Warmer temperatures favour the NH3 gas side of the equilibrium balance with NH4+, so the hotter it is, the greater the potential there is for nitrogen loss.
(c) Moisture. If the soil is dry and humidity is low then surface-applied AS will not dissolve and the potential for loss is low. If there is enough moisture to dissolve the fertiliser but not wash it into the soil, then the potential for losses is high – where pH and other conditions favour accumulation of NH3 gas in the soil water. Rainfall or irrigation is needed to transport the dissolved NH4+ into the soil where it can be adsorbed, nitrified (converted to nitrate) and/or taken up by plant roots. As the soil dries out, dissolved NH3 gas is concentrated in the remaining soil water solution increasing the potential for loss.
(d) Calcium carbonate (lime) content of the soil. NH4+ cations from AS fertiliser reacts directly with calcium carbonate (CaCO3) to produce ammonium bicarbonate or ammonium carbonate, both of which are unstable and rapidly break down to produce NH3 gas, CO2 gas, water and calcium sulphate. NH3 volatilisation has been directly related to the amount of CaCO3 present in the soil at least up to 12.5%.
(e) Soil clay content / cation exchange capacity (CEC). In soils, NH4+ can be adsorbed on the cation exchange surfaces of clay minerals, iron oxides and organic matter. Adsorption to these surfaces will draw down the NH4+ in the solution, so more dissolved NH3 gas will convert back to NH4+ to keep the same equilibrium – and so lower the total pool of NH3 gas available for loss. Therefore, under the same conditions, lighter soils will volatilise more than heavier soils with a higher CEC. NH4+ can also be fixed within the lattice structures of vermiculite and illite clay minerals from where it is only slowly available.
(f) Soil buffer capacity. In the NH4+/NH3 equilibrium equation, a hydrogen (H+) ion is produced with each NH3 gas molecule. H+ ions are what we measure as acidity. In soils with a low capacity to buffer increases in acidity, volatilisation will stop after the H+ released has lowered the pH enough that the equilibrium is again dominated by NH4+ (moves the equilibrium to the left in Figure 1). However, the alkaline soils of northwest NSW, particularly those with calcium carbonate present, often have high pH buffering capacities, so the H+ produced is absorbed (buffered) by the soil and the soil pH does not become more acidic so volatilisation continues. Acidity may develop in a localised area if the NH4+ is concentrated, as is around a fertiliser granule as opposed to a fine crystalline form or as a solution.
(g) Biological activity. NH4+ in the soil solution is subject to bacterial oxidation to form nitrate. This is the process of nitrification which depletes the soil solution of NH4+. To maintain the equilibrium balance, more dissolved NH3 is converted to NH4+ and so the total NH3 gas available for loss is decreased. Plant uptake also depletes the NH4+ pool.
(ii) Factors affecting the actual Volatilisation process
(a) Wind. Wind replaces air near the surface that has a higher partial NH3 pressure (from volatilisation) with fresh air having a lower (more normal) partial NH3 pressure. This rapid air replacement keeps a larger differential in partial pressures between the soil surface and the air above it, which drives further volatilisation to occur.
(b) Moisture. NH3 gas can volatilise without moisture loss from soil, but is likely to increase as soils dry because this increases the concentration of dissolved NH3 gas in the remaining soil water.
(c) Depth of fertiliser placement. NH3 gas must travel to the surface before it can be lost from the soil, so if it is incorporated rather than surface applied, losses are greatly reduced. NH3 gas diffusing through soil pores can be adsorbed by the surfaces of surrounding soil particles before it reaches the surface.
(d) Crop canopy. Even if NH3 gas has volatilised from the soil into the air, some of it may be re-absorbed by an actively growing crop canopy. Whether this happens will depend on whether the concentration of NH3 gas in the air is above that particular species’ “NH3 compensation point”. A crop canopy can also slow the wind speed across soil surface thus reducing the differential driving the volatilisation process.
(e) Residue management. Residues can be a physical barrier between soil and topspread fertiliser and may maintain a humid micro-environment that can dissolve fertiliser granules. For urea this can be doubly detrimental as the dissolved fertiliser solution is kept apart from the soil but kept in close proximity to the urease enzyme (on residue surfaces) which speeds the conversion of urea to NH3 gas.
Northwest NSW soil investigations
The factors and processes that contribute to the risk of nitrogen loss through volatilisation are well known. What is not known for much of the northwest NSW grains region are the levels and interactions of some of these factors, such as free lime content and pH buffer capacity, and the potential volatilisation losses that may result from different levels of these factors. This was one aim of our research. Singh et al. (2003) found that CaCO3 contents ranged from 0-8.5% with an average of 4.1% in 258 surface (0-10 cm) soils in irrigated cotton-growing areas of northwest NSW (lower Namoi, Macintyre and Gwydir River valleys). They also measured pH-buffering capacity in these soils, finding them generally high and variable, but not related to clay %, organic carbon, exchange sodium %, pH or calcium carbonate content.
We tested over 200 surface soil samples from previous dryland cropping projects in northern NSW for chemical properties that may play an important role in N volatilisation as discussed above. CaCO3 levels were generally lower than the results of Singh et al. (2003), with an average of 0.6% CaCO3 by weight (range: 0.0% to 6.4%). CaCO3 content was related to soil pH, which is to be expected as lime precipitates in soils at pHw between 7 and 8.5. Concentrations of more than 1% CaCO3 were only found in soils with pHw >8.1. We tested all samples with a pHw>7 for their capacity to buffer changes in pH back to 7 (pHBC) as this is where the risk of nitrogen volatilisation ceases (Figure 1). Percent CaCO3 in the soil was an excellent predictor of pH buffering capacity – CaCO3 explained 84% of the variation in pHBC results. Buffering capacity increased dramatically as CaCO3 increased above 0.5% of the soil.
Incubation studies
We incubated several vertosols (cracking clay soils) from northwest NSW cropping paddocks with surface-applied nitrogen fertilisers in closed containers. Each container was kept at constant temperature and had an internal acid trap to “drive” the volatilisation process and trap any NH3 volatilised into the air above the soil. The aim was to gauge the influence of soil properties, moisture content, and fertiliser products on volatilisation under controlled conditions. Volatilisation results from these incubations are useful for comparing relative differences between soils and treatments, but are not valid estimates of losses in field situations.
Preliminary experiments compared several soils with both high and low volatilisation potential, based on their soil properties. We found that, using surface-applied AS led to negligible losses from soils with nil CaCO3, neutral pH, low buffering capacity and moderate-high CEC (26-66 cmol+/kg) – regardless of moisture content. Losses from soils with 6-7% CaCO3, alkaline pH, high pH buffering capacity, and moderate-high CEC (30-72 cmol+/kg) were between 10 and 100 times greater, with soil at 40% water-holding capacity losing more than that incubated at drier or wetter moisture contents. The atmosphere inside all but the driest incubation containers was humid enough to dissolve the fertiliser granules, with those wetter than 40% allowing the dissolved nitrogen in solution to diffuse into the soil and adsorb to the soil surfaces. Mixtures of soils with nil and 7% CaCO3 gave volatilisation losses proportional to the amount of CaCO3 in the mixture.
A comparison of volatilisation losses from a range of nitrogen fertiliser products using a 7% CaCO3 soil incubated at 40% water-holding capacity is shown in Figure 2. Rather than report in units of actual kg N/ha lost, which are not valid field estimates, results are given relative to the total N loss from ordinary urea after 18 days of measurement.
Figure 2. Cumulative volatilisation loss of nitrogen (N) applied as fertiliser to the surface of an alkaline soil containing 7% CaCO3, relative to the total N loss from urea after 18 days. All products were applied to the soil surface at 100 kg N/ha. Urea ammonium nitrate and ammonium nitrate were applied as liquids, all others as solids. All points are means of three replications of each treatment with standard error bars showing the variation.
Nitrogen losses from crystalline ammonium sulphate (AS) were nearly twice that of urea and occurred at a faster rate to losses from urea. Greatest AS loss occurred in the early stages of incubation whereas urea losses began only after a few days incubation, after the urea hydrolysis step where urea is converted to NH4+. Preliminary experiments showed no difference between granular and crystalline AS.
Urea coated with a urease inhibitor showed very low volatilisation during the incubation period as the urea was chemically prevented from hydrolysing into the ammonium/ammonia form.
Urea with nitrification inhibitor was not prevented from hydrolysing from urea to ammonia, but was prevented from being nitrified into nitrate. N volatilisation loss from this product followed a similar pattern to unmodified urea for the first week, but continued to be lost at a steady rate after losses from the unmodified urea had ceased, presumably due to nitrification drawing down the total NH4+/NH3 pool.
Urea coated with an organic complex showed no difference from ordinary urea in N volatilisation loss measured in these incubations.
N volatilisation loss from the zeolite-coated urea was about double that of the ordinary urea in our incubations. It may be that the zeolite, a clay mineral, absorbed the ammonium from the dissolved urea solution and kept some of it from contact with the soil until it was lost to the acid trap above the soil.
Losses from the two liquid products were less than the solids as the liquids moved directly into contact with soil surfaces when applied as droplets from a shower rose. Losses from UAN were slightly higher as this product incorporates both ammonium and urea forms (which converted to yield more ammonium), hence a greater proportion of the product potentially available to be volatilised
Conclusions
We don’t know the magnitude of actual volatilisation losses from ammonium sulphate topdressed either pre or post-sowing in northwest NSW. However, the risk factors are well-enough known to predict that significant losses will occur where soils are alkaline, moist, warm, are light to medium textured, and especially where they have free lime at the surface. Evidence from the literature suggests that N losses of 25% or more may occur. Incorporation of N fertilisers in such circumstances will greatly reduce the risk of NH3 gas loss. If you know your soil is alkaline, you can easily test for the presence of CaCO3 by adding droplets of dilute acid to soil or to any visible white “nodules” and watching to see if they “fizz” (bubbles of CO2 are rapidly produced as the acid dissolves the CaCO3).
Further research support is needed to get real-world measurements of nitrogen volatilisation from different products, application methods, and conditions as it happens in the field. NH3 volatilisation can constitute a potential economic loss to the farmer, and, although NH3 is not a greenhouse-warming gas like nitrous oxide (N2O), it does contribute to greenhouse gas emissions calculations through re-deposition of the volatilised NH3 to the soil and subsequent loss as N2O.
Reference
Singh B, Odeh IOA, McBratney AB (2003) Acid buffering capacity and potential acidification of cotton soils in northern New South Wales. Australian Journal of Soil Research 41, 875-888.
Acknowledgements
Many thanks to Jan Hosking for lab assistance, also Pat Mortell and Adam Perfrement for soil collection. NSW DPI, NGA and GRDC fund the current Eastern Farming Systems Project in northern NSW. The soil samples reanalysed in this study came from several previous research and extension projects funded by GRDC, ACIAR and NHT. We acknowledge the efforts of those who collected the samples for these projects and to Ian Daniells who kindly allowed us use of the other soil data collected at the time.
Contact details
Graeme Schwenke
Ph: 02 67631137
Fx: 02 67631222
Email: graeme.schwenke@dpi.nsw.gov.au
|
Guy McMullen
Ph: 02 67631155
Fx: 02 67631222
Email: guy.mcmullen@dpi.nsw.gov.au
|
Was this page helpful?
YOUR FEEDBACK