Understanding heat impacts on wheat to breed future tolerance
Take home messages
- Short periods of heat stress have different impacts on yield and quality components in wheat depending when the stress occurs during development. Different genes can give tolerance for the different stages/effects.
- In greenhouse/chamber screens, heat tolerance for thousand kernel weight (1000-kw) stability was correlated with staygreen. The Sr2 stem rust resistance locus partly accounted for this relationship, where heat sensitivity of grain weight and chlorophyll was coupled with rust resistance.
- Heat tolerant hexaploid and tetraploid wheat genotypes were identified in greenhouse/growth chamber experiments and in irrigated field trials in southern NSW where delayed sowing was used to impose heat stress. These potential tolerance sources need to be validated in rainfed field trials sown at the normal time.
- The field trials also suggested the International Maize and Wheat Improvement Centre (CIMMYT) and Australian genotypes are likely to yield best under irrigation in NSW. However, production of premium grain quality under irrigation seems most feasible for hexaploid wheat, combined with timely sowing and good N management.
Background
Heat waves of greater than 30 °C for approximately three days commonly occur during wheat reproductive development in southern Australia, reducing yield (grain number and size effects) and altering processing and end-use quality of the grain. Specific windows of development are sensitive to the different effects (Figure 1). Coupled with the irregular nature of heat stress events in the field, these developmental sensitivities hamper selection of heat tolerance by breeders. Our main strategy to tackle this problem is to develop DNA markers to assist breeders to select new heat tolerant varieties. This firstly requires the identification of wheat genotypes contrasting for heat responses, which can subsequently be used as parents in genetic studies.
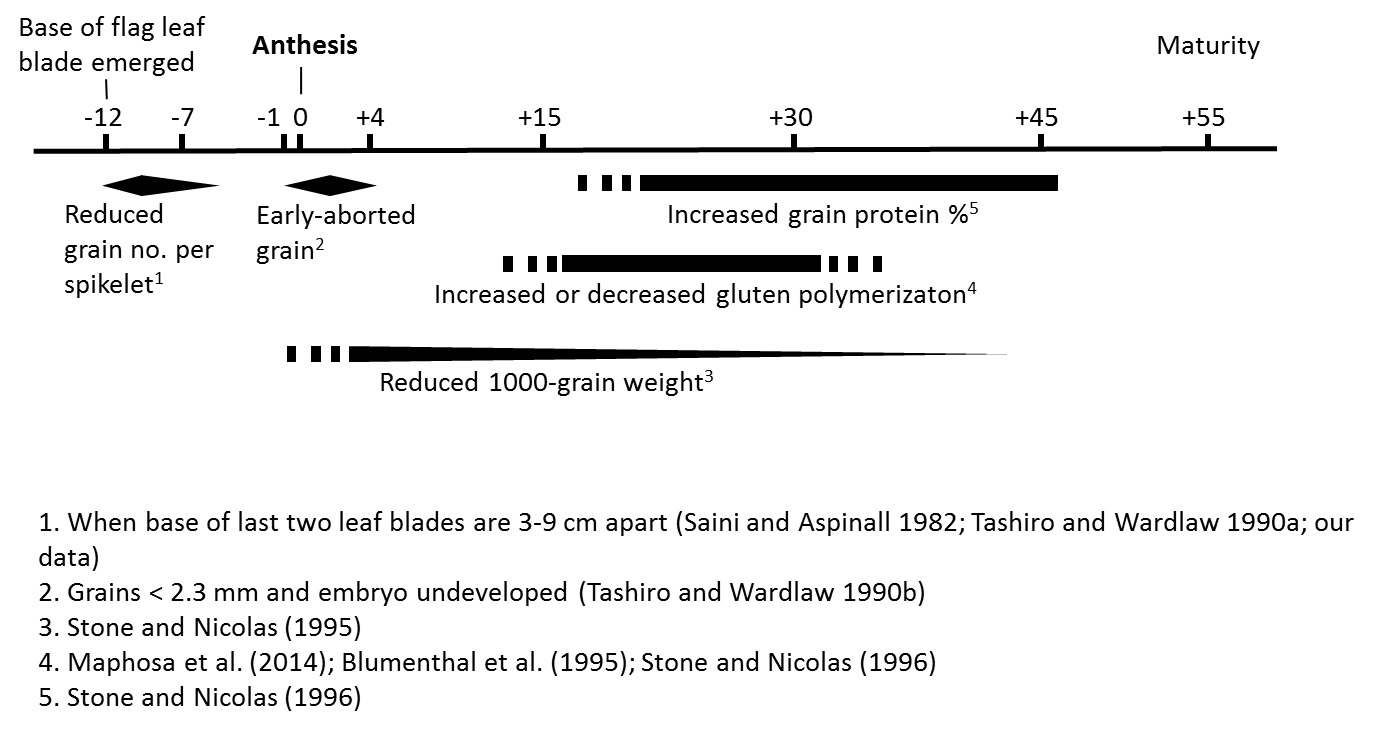
Figure 1. Sensitivities of various wheat developmental stages to the effects of short heat treatments, as defined by our own and others’ controlled environment studies. Typical treatments were at 30 to 40 °C during the day cycle for 2 to 5 days. Filled shapes indicate timing/intensity of effects on traits measured at maturity. Dotted portions indicate uncertain boundaries. Horizontal scale shows days before/after anthesis.
Greenhouse/chamber screening
Methods
Plants were grown one to a pot (8cm wide, 18cm deep) under non-stress conditions in a greenhouse, then heat-treated in a growth chamber set at 37/27 °C day/night for 3 days, transferred back to the greenhouse and the main stem scored at maturity. Each plant to be heat treated was moved to the chamber when its main stem reached the target developmental stage, based on the information in Figure 1. That is, either when the base of the flag leaf blade was 3 to 9cm emerged for assessing floret fertility effects or at 10 days after anthesis for assessing 1000-kw effects. Unheated control plants were included for comparison. A randomized block split-plot design was used with 6 to 10 replicates, with the heat-treated and control plant for each genotype being neighbours within each block (replicate). Although pots were placed in shallow trays of water during the heat treatment, plants appeared partly wilted during the day cycle due to the high evaporative demand (relative humidity was 45%; vapour pressure deficit (VPD) = 3.45 kPa).
Results and discussion
Hexaploid and tetraploid genotypes showed a wide variation in heat induced floret sterility after a short treatment applied prior to heading, ranging to almost no reduction in fertility, to almost complete loss of fertility (Table 1).
Table 1: Floret fertility of wheat genotypes after a 3d heat treatment applied when the base of the flag leaf blade was 3 to 9cm emerged, in greenhouse/chamber assays1.
Hexaploid genotype | Ave. % fertility under heat stress | No. of times tested | Tetraploid genotype | Ave. % fertility under heat stress | No. of times tested |
---|---|---|---|---|---|
Tasman | 95 | 2 | Hyperno | 74 | 2 |
Sunco | 93 | 5 | Saintly | 70 | 2 |
Sunstar | 93 | 2 | Tamaroi | 66 | 1 |
Excalibur | 90 | 3 | Yallaroi | 60 | 2 |
Young | 90 | 4 | Kamilaroi | 56 | 1 |
Waagan | 88 | 3 | Kronos | 43 | 2 |
Janz | 85 | 2 | WID 802 | 32 | 1 |
Gladius | 84 | 3 | Tjilkuri | 27 | 1 |
Kukri | 72 | 3 | Yawa | 26 | 1 |
EGA Stampede | 70 | 2 | Kamilaroi | 25 | 1 |
Krichauff | 68 | 2 | Kalka | 22 | 1 |
EGA Gregory | 67 | 2 | Jandaroi | 9 | 1 |
Sokoll | 61 | 2 | |||
GBA Hunter | 56 | 2 | |||
Drysdale | 55 | 3 | |||
Reeves | 46 | 2 | |||
Cadoux | 45 | 4 | |||
Kauz | 39 | 2 | |||
Westonia | 9 | 4 |
1Genotypes are ordered by tolerance. Based on the main stem, bottom two floret positions, in spikelets from the lower two-thirds of the spike (2 grains per spikelet = 100% fertility). Fertility in non-heated control plants was greater than 90% (not shown). Based on a subjective impression of reproducibility, genotypes differing by less than 20% fertility in this table cannot be reliably discriminated for tolerance.
Screening results for 1000-kw effects of heat, obtained using a short heat stress applied at 10 days after anthesis, are presented here only for hexaploids (Table 2). Reductions in 1000-kw due to the treatment varied from essentially zero to approximately 50% relative to control in the most sensitive varieties (data not shown). Interestingly, Westonia was ranked the most tolerant for 1000-kw effects, but was very sensitive to heat induced floret sterility (Table 1), suggesting that different genes provide tolerance to the grain number versus size effects of heat.
Table 2. Tolerance indexes of wheat genotypes for their ability to maintain 1000-kw after a 3d heat treatment applied 10 days after anthesis, in greenhouse/chamber assays1.
Genotype | 1000-kw tol. index (g) | n | Genotype | 1000-kw tol. index (g) | n | Genotype | 1000-kw tol. index (g) | n |
---|---|---|---|---|---|---|---|---|
Westonia | 11.0 | 2 | Trident | 3.5 | 1 | Frame | -2.4 | 2 |
Egret | 10.5 | 2 | Cook | 3.5 | 1 | Cranbrook | -2.4 | 1 |
EGA Eaglehawk | 10.3 | 1 | Avocet | 3.1 | 1 | Fang | -2.6 | 2 |
Young | 8.4 | 2 | Molineux | 2.8 | 1 | Catalina | -2.6 | 1 |
Waagan | 8.1 | 2 | Berkut | 1.8 | 1 | Millewa | -2.7 | 2 |
RAC875 | 7.8 | 1 | Mendos | 1.4 | 1 | Calingiri | -3.4 | 1 |
Gladius | 7.5 | 3 | EGA 2248 | 1.1 | 1 | Halberd | -4.7 | 1 |
Sunco | 5.5 | 2 | Sunstar | 0.7 | 1 | Cadoux | -5.3 | 2 |
Correll | 5.3 | 2 | EGA Bonnie Rock | 0.7 | 2 | Sunvex | -5.4 | 1 |
Chara | 5.0 | 1 | Kukri | 0.2 | 2 | Drysdale | -6.7 | 4 |
Tamarin Rock | 4.8 | 1 | Sokoll | 0.2 | 2 | CD87 | -6.8 | 1 |
WW2449 | 4.3 | 1 | Ajana | 0.1 | 1 | Axe | -6.8 | 1 |
Gascoigne | 4.1 | 1 | Vorobey | 0.1 | 3 | H45 | -7.0 | 3 |
Barham | 4.0 | 1 | Excaliber | -0.4 | 3 | Kite | -8.1 | 1 |
EGA Blanco | 4.0 | 1 | Wyalkatchem | -0.6 | 1 | Reeves | -8.1 | 2 |
Janz | 4.0 | 1 | Lincoln | -0.9 | 1 | Tasman | -8.3 | 2 |
Krichauff | 3.9 | 2 | Aroona | -1.7 | 1 | Wyuna | -10.7 | 1 |
King Rock | 3.9 | 2 | Hartog | -2.3 | 2 | Crusader | -13.5 | 1 |
H46 | 3.5 | 1 |
1Genotypes are ordered by tolerance, with larger values indicating greater tolerance. Tolerance index is the deviation of the value under heat relative to the value expected based on the linear regression between heat versus control 1000-kw values across all genotypes. This gives a measure of stability which is largely independent of 1000-kw in control. n = number of screening experiments in which a genotype was included; averages of tolerance indexes across experiments are shown. Based on a subjective impression of reproducibility, genotypes differing by less than 10g for the heat tolerance index in this table cannot be reliably discriminated for tolerance.
Since cvs. Drysdale and Waagan contrasted for the effects of heat on both floret fertility and 1000-kw, a Drysdale x Waagan doubled-haploid population was used to investigate the genetics of tolerance. The strongest determinant of 1000-kw tolerance was located at or near the Sr2 rust resistance locus on chromosome 3B, with the tendency to lose grain weight under heat (from Drysdale) being coupled with enhanced heat-induced leaf chlorophyll loss (Shirdelmoghanloo et al. 2016a) and rust resistance (our unpublished data). This was consistent with a previous report that Sr2 confers a heat-dependent chlorosis phenotype in seedlings (Brown 1997). It raises the possibility that varieties carrying Sr2 stem rust resistance may be more prone to grain weight loss under heat stress, although this still needs to be tested in the field.
A second (weaker) 1000-kw tolerance locus was detected on chromosome 6B. Here, heat stability of grain size and chlorophyll were again coupled (Shirdelmoghanloo et al. 2016a), however we are not aware of any rust resistance gene in that region. A positive correlation between chlorophyll and grain size stability under heat was also observed across 36 wheat genotypes (Pearson’s correlation test, p < 0.01; Shirdelmoghanloo et al. 2016b). Hence, staygreen appeared to be associated with mechanisms of grain filling heat tolerance and this was sometimes a result of variation at the Sr2, locus, but not always.
The Drysdale x Waagan population was also assessed for heat induced floret sterility. A single strong tolerance effect was detected on chromosome 2B. As this locus was separate from the 1000-kw tolerance loci on 3B or 6B, it confirmed that genetic control of tolerance to the effects of heat on grain size and grain number could be independent.
Field trials
Methods
Field trials were conducted in southern NSW, at Leeton in 2011 and Wagga Wagga in 2012. Yield plots (6 rows spaced at 30cm x 6m) were sown at two times (first week of June and first week of August), with genotypes sown in randomly located duplicates for each sowing date. Standard practice was followed for fertilisation and chemical control of fungal diseases. In the first 10 days of grain filling, plots from the late sowings experienced greater average daily maximum temperatures than in the timely sowings (28.8 versus 21.4 °C at Leeton and 26.8 versus 20.3 °C at Wagga Wagga) and more days over 30 °C (average 4.9 versus 0.5 at Leeton and 3.0 versus 0.2 at Wagga Wagga), although daily maximum temperatures remained below 35 oC. Hence, the August sown plots experienced more heat stress than the June sown plots. Flood irrigation was applied to plots sown at both times with the aim of minimizing drought stress; however some wilting was observed on the hottest days. Data are reported for 104 hexaploid wheat genotypes and 13 tetraploid wheat varieties. The former included new and old Australian varieties, breeding lines and genotypes from overseas (mostly CIMMYT origin). High Temperature Wheat Yield Trial (HTWYT) lines were breeding lines from CIMMYT’s High Temperature Wheat Yield Trials. Richard Trethowan (RT) lines were generated by Richard Trethowan from CIMMYT germplasm by repeated selection for high 1000-kw under terminal drought/heat stress. Genotypes flowered within a 15 day interval for each sowing.
Results and discussion
Performance in the June (‘timely’) sowings could be of interest for irrigated cropping (Tables 3 and 4). Yields were up to 9t/ha at Leeton, and 6.6t/ha at Wagga Wagga. On average, yields were higher in the durums than in the hexaploids (by 1.1t/ha at Leeton and 0.3t/ha at Wagga Wagga). An approximate 1t/ha yield advantage of durums over hexaploid wheats under irrigated conditions has also been reported for trials in the Northern wheat zone (Sykes 2012). However, the highest individual yields were achieved by hexaploid genotypes, mostly of CIMMYT origin or parentage (HTWYT 001, HTWYT 002 and HTWYT 009, GBA Hunter, Zebu).
1000-kw was generally high, more so in the durums (average 47 versus 40g in the hexaploids) and they also had lower percentage screenings (0.6 versus 2.3% in the hexaploids). Average test weight (approximately 81kg/hL) was above the target of 76kg/hL for prime quality. On average, grain protein was around the target level for prime quality (approximately 13%), and of the 15 varieties classified as Australian Prime Hard (APH), six averaged greater than 13% across trials. This was consistent with previous reports that irrigation decreases grain protein; however, good nitrogen (N) management can bring grain protein of irrigated durum to high levels (Sissons et al. 2014).
Table 3. Performance of hexaploid wheat genotypes with June sowings, in irrigated field trials, Leeton 2011 and Wagga Wagga 20121.
Genotype | Yield, Leeton (t/ha) | Yield, Wagga Wagga (t/ha) | Yield, ave. both trials (t/ha) | Yield, kw, ave. both trials (g) | % Screenings, ave. both trials | Test weight, ave. both sites (kg/hL) | % Grain protein, ave. both trials |
---|---|---|---|---|---|---|---|
HTWYT 002 | 9.0 | 6.6 | 7.8 | 41 | 0.4 | 81 | 12.6 |
HTWYT 001 | 8.5 | 6.0 | 7.2 | 42 | 0.9 | 83 | 12.5 |
GBA Hunter | 8.4 | 5.9 | 7.2 | 38 | 2.7 | 82 | 12.9 |
HTWYT 009 | 8.2 | 6.0 | 7.1 | 38 | 3.7 | 84 | 13.3 |
Zebu | 8.0 | 5.9 | 7.0 | 38 | 2.7 | 82 | 12.8 |
Bolac | 7.4 | 6.1 | 6.8 | 34 | 4.6 | 80 | 12.5 |
RT812 | 8.1 | 5.4 | 6.8 | 43 | 1.3 | 82 | 12.1 |
EGA Stampede | 8.0 | 5.5 | 6.7 | 40 | 3.1 | 81 | 11.7 |
6HRWSN98 | 7.7 | 5.5 | 6.6 | 36 | 1.0 | 83 | 13.3 |
Seri M82 | 7.4 | 5.9 | 6.6 | 43 | 0.7 | 81 | 12.1 |
Lincoln | 7.2 | 6.0 | 6.6 | 42 | 1.9 | 82 | 12.8 |
Axe | 7.5 | 5.7 | 6.6 | 39 | 0.6 | 82 | 13.0 |
Sentinel | 8.1 | 5.1 | 6.6 | 44 | 0.6 | 82 | 12.4 |
Kauz | 7.1 | 5.9 | 6.5 | 37 | 1.8 | 82 | 13.3 |
Espada | 7.0 | 5.8 | 6.4 | 40 | 0.8 | 80 | 13.0 |
Scout | 7.2 | 5.6 | 6.4 | 40 | 3.9 | 84 | 12.3 |
Gascoigne | 6.5 | 6.0 | 6.3 | 45 | 0.9 | 85 | 13.1 |
Buckley | 6.9 | 5.5 | 6.2 | 39 | 0.8 | 78 | 11.1 |
Janz | 6.9 | 5.6 | 6.2 | 38 | 2.4 | 82 | 12.3 |
Livingston | 7.1 | 5.3 | 6.2 | 40 | 2.4 | 82 | 13.6 |
EGA Wills | 6.6 | 5.7 | 6.1 | 40 | 1.4 | 81 | 12.3 |
Giles | 6.8 | 5.4 | 6.1 | 37 | 3.6 | 82 | 11.8 |
Ventura | 6.6 | 5.5 | 6.0 | 39 | 4.5 | 81 | 13.5 |
Correll | 6.5 | 5.4 | 6.0 | 41 | 2.9 | 78 | 13.5 |
Young | 6.5 | 5.4 | 5.9 | 34 | 6.0 | 80 | 13.4 |
Hartog | 6.8 | 5.0 | 5.9 | 38 | 2.9 | 82 | 13.2 |
Guardian | 6.9 | 4.9 | 5.9 | 36 | 5.3 | 82 | 12.4 |
Gladius | 6.7 | 5.0 | 5.9 | 42 | 1.8 | 78 | 13.0 |
Waagan | 7.1 | 4.5 | 5.8 | 37 | 3.2 | 80 | 12.7 |
Catalina | 5.8 | 5.8 | 5.8 | 40 | 3.3 | 82 | 13.0 |
Yitpi | 6.5 | 5.1 | 5.8 | 40 | 2.4 | 79 | 13.1 |
EGA Gregory | 6.4 | 5.2 | 5.8 | 41 | 2.0 | 82 | 12.3 |
Sunstate | 6.6 | 4.8 | 5.7 | 39 | 2.7 | 83 | 13.4 |
Sunelg | 6.7 | 4.7 | 5.7 | 38 | 4.2 | 82 | 14.0 |
Peake | 6.3 | 4.9 | 5.6 | 37 | 4.2 | 81 | 12.5 |
Cunningham | 6.3 | 4.9 | 5.6 | 37 | 2.2 | 83 | 13.0 |
Kennedy | 6.2 | 5.0 | 5.6 | 33 | 5.2 | 82 | 12.8 |
Dakota | 5.7 | 5.4 | 5.5 | 36 | 4.2 | 82 | 12.2 |
Ellison | 6.1 | 5.0 | 5.5 | 43 | 2.2 | 83 | 14.4 |
Cranbrook | 6.2 | 4.8 | 5.5 | 40 | 2.0 | 80 | 12.6 |
Diamondbird | 6.1 | 4.9 | 5.5 | 39 | 2.8 | 81 | 12.7 |
Clearfield JNZ | 6.1 | 4.9 | 5.5 | 38 | 1.1 | 82 | 12.2 |
EGA Bounty | 6.4 | 4.6 | 5.5 | 36 | 3.0 | 82 | 12.6 |
Drysdale | 6.4 | 4.6 | 5.5 | 39 | 3.5 | 82 | 12.7 |
Derrimut | 5.9 | 5.1 | 5.5 | 35 | 3.1 | 83 | 11.9 |
Oxley | 6.2 | 4.7 | 5.5 | 36 | 5.9 | 79 | 13.2 |
Magenta | 6.3 | 4.2 | 5.3 | 40 | 5.5 | 81 | 12.7 |
Banks | 6.0 | 4.5 | 5.2 | 36 | 1.4 | 82 | 12.5 |
Sunvex | 6.1 | 4.2 | 5.1 | 38 | 1.1 | 82 | 13.7 |
Fang | 6.2 | 3.8 | 5.0 | 36 | 5.5 | 82 | 12.8 |
Zippy | 6.0 | 3.9 | 5.0 | 36 | 0.8 | 82 | 14.1 |
Sunstar | 5.8 | 4.1 | 4.9 | 33 | 2.1 | 80 | 12.9 |
Molineux | 5.7 | 4.1 | 4.9 | 36 | 4.2 | 81 | 12.7 |
Tamarin Rock | 5.4 | 4.5 | 4.9 | 42 | 1.7 | 80 | 13.0 |
Trident | 5.2 | 4.3 | 4.8 | 36 | 3.3 | 80 | 13.1 |
Carnamah | 4.9 | 4.5 | 4.7 | 39 | 1.4 | 81 | 13.0 |
Crusader | 5.3 | 4.1 | 4.7 | 33 | 3.7 | 82 | 13.8 |
Excalibur | 5.4 | 3.9 | 4.7 | 38 | 3.8 | 77 | 13.5 |
Mace | 5.0 | 4.2 | 4.6 | 39 | 3.3 | 81 | 13.0 |
Chara | 5.1 | 3.8 | 4.5 | 36 | 6.0 | 80 | 12.7 |
Cadoux | 4.6 | 4.4 | 4.5 | 38 | 0.6 | 80 | 12.1 |
Calingiri | 4.5 | 4.1 | 4.3 | 41 | 2.9 | 80 | 12.6 |
Barham | 4.5 | 4.0 | 4.3 | 35 | 2.7 | 76 | 11.9 |
Wyalkatchem | 3.6 | 4.0 | 3.8 | 37 | 2.2 | 79 | 13.8 |
Mendos | 3.4 | 3.5 | 3.5 | 40 | 0.5 | 76 | 14.8 |
Kings White | 3.2 | 3.4 | 3.3 | 45 | 0.5 | 80 | 15.2 |
Average all hexaploids | 6.4 | 4.8 | 5.6 | 40 | 2.3 | 81 | 13.0 |
1Non-Australian genotypes are shown only if their yields averaged greater than 6.5t/ha across trials. Genotypes are ordered by average yield across both trials. Yield and 1000-kw figures were adjusted to account for spatial factors and flowering time, while figures for other traits are raw means.
The highest yielding durums were Caparoi and the recent releases Yawa and Tjilkuri. In the durums where a high proportion of hard vitreous kernels (HVK) is desired (HVK greater than 80%), %HVK was very low at Leeton (average 50%), but somewhat better at Wagga Wagga (average 74%) (data not shown). Low %HVK has previously been noted in irrigated durum and may pose a barrier to marketability (Sisssons et al. 2014).
Table 4. Performance of tetraploid wheat varieties with June sowings, in irrigated field trials, Leeton 2011 and Wagga Wagga 20121.
Genotype | Yield, Leeton (t/ha) | Yield, Wagga Wagga (t/ha) | Yield, ave. both trials (t/ha) | 1000-kw, ave. both trials (g) | % Screenings, ave. both trials | Test weight, ave. both sites (kg/hL) | % Grain protein, ave. both trials |
---|---|---|---|---|---|---|---|
Tjilkuri | 8.0 | 5.7 | 6.9 | 46 | 0.7 | 82 | 11.6 |
Yawa | 7.9 | 5.7 | 6.8 | 47 | 0.4 | 83 | 12.5 |
Caparoi | 7.8 | 5.7 | 6.8 | 49 | 0.2 | 84 | 12.8 |
Tamaroi | 7.5 | 5.4 | 6.4 | 49 | 0.6 | 83 | 13.8 |
Atil C2000 | 7.5 | 5.1 | 6.3 | 44 | 0.3 | 83 | 11.6 |
Hyperno | 7.3 | 5.3 | 6.3 | 46 | 1.2 | 83 | 11.8 |
Kronos | 7.4 | 5.1 | 6.3 | 50 | 0.2 | 80 | 13.7 |
WID 802 | 7.3 | 5.1 | 6.2 | 47 | 1.1 | 82 | 12.7 |
Saintly | 7.2 | 5.0 | 6.1 | 44 | 2.1 | 83 | 12.7 |
Kalka | 7.4 | 4.6 | 6.0 | 48 | 0.1 | 84 | 12.4 |
Bellaroi | 7.3 | 4.7 | 6.0 | 47 | 0.2 | 81 | 13.4 |
Jandaroi | 7.1 | 4.5 | 5.8 | 48 | 0.1 | 82 | 14.0 |
Durati | 6.8 | 4.8 | 5.8 | 49 | 0.3 | 81 | 14.3 |
Average | 7.4 | 5.1 | 6.3 | 47 | 0.6 | 82 | 12.9 |
1All 13 varieties trialled are shown. Other details are the same as for Table 3.
Relative to the timely sowings, the late sowings decreased the time from sowing to anthesis, grain yield, 1000-kw and test weight and increased % screenings, % grain protein and %HVK (Table 5). As for the timely sowings, none of the Australian hexaploid or durum varieties in the late sowings consistently (in both trials) met the 13% protein, 76kg/hL test weight and less than 5% screenings targets for the premium classification. In the late sowings, HypernoA met the 80% HVK level required for the premium durum classification, but it produced high screenings (6-9%) (data not shown).
Floret fertility (grain set per spikelet) probably wasn’t affected by late sowing due to insufficiently high temperatures prior to anthesis in these trials; however, tiller density and/or spikelet number per spike effects were likely to have been responsible for yield loss not otherwise accounted for by responses in 1000-kw (data not shown).
Table 5. Average responses of wheat genotypes to late sowing, expressed as the difference between late vs. timely sown values.
Trial | Class | Time to anthesis (d) | Yield (t/ha) | 1000-kw (g) | % screenings | Test weight (kg/hL) | % grain protein | % HVK |
---|---|---|---|---|---|---|---|---|
Leeton 2011 | Hexaploid | -30.6 | -2.4 | -8.1 | 3.7 | -2.1 | 0.9 | nd |
Tetraploid | -33.7 | -2.7 | -11.5 | 4.4 | -4.1 | 1.6 | 27 | |
Wagga Wagga 2012 | Hexaploid | -38.3 | -1,3 | -7.3 | 4.8 | -5.2 | 1.1 | nd |
Tetraploid | -42.3 | -2.1 | -8.4 | 1.2 | -5.1 | 1.9 | 9.1 |
Among the hexaploid wheats, genotypes whose yield was decreased least by late sowing included CIMMYT lines (HTWYT 012 and HTWYT 001, Kauz, RT812 and Tepoca 89), and the NSW variety WaaganA, which was derived by a cross between Janz and a CIMMYT line (Table 6). Overall, the breeding lines HTWYT 001 and RT812 showed the best balance of yield stability with late sowing, and high yield with timely sowing. HTWYT 001 did develop high screenings in the late sowings, but this should not be a problem if it is used as for feed.
Table 6. Performance ranking in August sowings relative to June sowings in hexaploid wheat genotypes, averaged across Leeton 2011 and Wagga Wagga 2012 irrigated field trials (lower values are more favourable in each case)1.
Genotype | Yield stability ranking | 1000-kw stability ranking | Increase in % screenings with later sowing | Test weight stability ranking | % grain protein gain ranking |
---|---|---|---|---|---|
HTWYT 012 | 4.5 | 17.0 | 1.9 | 29 | 99 |
Waagan | 11.0 | 96.0 | 1.8 | 45.5 | 81 |
Kauz | 13.0 | 34.5 | 0.5 | 12.5 | 68.5 |
RT812 | 15.0 | 70.5 | 0.4 | 48.5 | 86 |
Tepoca 89 | 17.5 | 49.5 | 2.3 | 64.5 | 25.5 |
HTWYT 001 | 20.5 | 35.0 | 7.7 | 9 | 71 |
Gascoigne | 20.5 | 83.5 | 3.6 | 40 | 41.5 |
RT425 | 22.5 | 52.0 | 2.2 | 15 | 83 |
Excalibur | 22.5 | 11.0 | 0.2 | 36.5 | 42 |
Correll | 24.0 | 13.5 | 2.1 | 87 | 39 |
Janz | 24.5 | 77.0 | 4.8 | 69.5 | 66 |
EGA Wills | 24.5 | 80.5 | 4.1 | 75.5 | 27 |
Peake | 25.5 | 62.0 | 0.6 | 51.5 | 88 |
Sunvex | 26.0 | 38.5 | 3.0 | 47.5 | 4.5 |
Diamondbird | 26.0 | 37.0 | 7.7 | 61 | 84 |
Chara | 26.5 | 44.5 | 7.0 | 70 | 31.5 |
Lincoln | 31.0 | 88.5 | 4.1 | 66.5 | 13 |
Gladius | 32.0 | 46.5 | 1.2 | 68 | 51 |
Fang | 34.5 | 51.0 | 4.6 | 24.5 | 39.5 |
Magenta | 35.5 | 77.0 | 5.7 | 87.5 | 7 |
Sunvale | 42.5 | 59.0 | 7.6 | 64.5 | 19.5 |
Barham | 43.5 | 46.5 | 2.0 | 84 | 74.5 |
EGA Stampede | 43.5 | 100.0 | 6.2 | 64 | 94 |
Cunningham | 44.5 | 54.0 | 10.4 | 39 | 20.5 |
Sunstate | 45.0 | 58.5 | 8.6 | 51.5 | 81.5 |
Yitpi | 46.0 | 32.5 | 4.3 | 69.5 | 56 |
Zebu | 47.0 | 51.5 | 0.8 | 58 | 21.5 |
Livingston | 47.0 | 78.0 | 2.1 | 47.5 | 49.5 |
Espada | 48.5 | 42.0 | 4.3 | 80 | 25.5 |
Ellison | 49.0 | 86.5 | 7.1 | 65 | 48.5 |
Sunstar | 49.0 | 43.5 | 5.3 | 84 | 30 |
GBA Hunter | 49.5 | 13.5 | 3.5 | 35 | 57.5 |
Scout | 51.0 | 61.5 | 3.4 | 22 | 61 |
Ventura | 51.5 | 37.0 | 5.8 | 74.5 | 63.5 |
Sentinel | 53.5 | 103.0 | 5.3 | 88.5 | 14 |
Tamarin Rock | 53.5 | 43.5 | 5.8 | 80 | 101.5 |
Zippy | 55.5 | 91.5 | 1.6 | 10 | 91.5 |
Molineux | 56.5 | 63.5 | 8.0 | 57 | 60 |
Mace | 57.0 | 33.5 | 4.6 | 35.5 | 97 |
Drysdale | 59.5 | 25.0 | 4.2 | 55 | 83 |
Catalina | 60.5 | 69.0 | 5.4 | 60.5 | 60.5 |
Dakota | 61.0 | 104.0 | 6.3 | 99.5 | 32 |
Clearfield JNZ | 61.5 | 59.0 | 5.9 | 70.5 | 37.5 |
Banks | 61.5 | 53.0 | 4.3 | 72.5 | 53.5 |
Axe | 62.0 | 85.0 | 6.0 | 69.5 | 42.5 |
Guardian | 66.0 | 55.5 | 9.4 | 53 | 50 |
Trident | 66.0 | 77.0 | 5.8 | 19 | 85 |
Oxley | 68.0 | 59.5 | 1.4 | 77.5 | 15.5 |
Kennedy | 69.5 | 67.5 | 12.2 | 75 | 42 |
Derrimut | 70.5 | 39.0 | 3.4 | 50.5 | 78 |
EGA Gregory | 72.0 | 81.0 | 8.2 | 85 | 16.5 |
Giles | 72.5 | 74.0 | 8.4 | 53.5 | 54 |
Hartog | 73.0 | 89.0 | 6.1 | 66 | 35.5 |
Young | 73.5 | 67.5 | 1.4 | 38.5 | 54 |
Sunleg | 73.5 | 17.5 | 7.7 | 86.5 | 20.5 |
Buckley | 74.0 | 99.0 | 3.1 | 98.5 | 54 |
Mendos | 75.0 | 4.0 | -0.3 | 45.5 | 42 |
Cranbrook | 76.0 | 85.0 | 1.4 | 94 | 69.5 |
Kings White | 78.0 | 4.5 | 0.1 | 9 | 44.5 |
Bolac | 83.5 | 80.5 | 9.4 | 87 | 5.5 |
EGA Bounty | 86.0 | 60.5 | 11.7 | 60.5 | 7.5 |
Crusader | 86.5 | 72.0 | 6.0 | 42.5 | 99 |
Carnamah | 94.0 | 96.5 | 7.3 | 64.5 | 66.5 |
Calingiri | 94.5 | 49.5 | 2.5 | 73.5 | 66.5 |
Cadoux | 95.5 | 55.5 | 6.3 | 59 | 66.5 |
Wyalkatchem | 96.0 | 22.5 | 0.5 | 38.5 | 62 |
1Non-Australian genotypes are shown only if they ranked in the top 25 for yield stability. In each trial, stability for yield, 1000-kw, test weight and % grain protein was defined as the deviation of the observed value in the late sowing from the expected value based on the linear regression of August vs. June sown values across all trialled hexaploid genotypes. This gives a measure of stability which is largely independent of performance per se in the June sowing. Yield and 1000-kw were adjusted for spatial factors and flowering time prior to the regression step. For % grain protein, the least stable lines were ranked first, as late sowing changed % grain protein in a desirable direction. The effect of late sowing for % screenings is represented as the per se increase in % screenings observed with late sowing vs. that obtained with timely sowing.
Among the Australian durums, Saintly, Hyperno and Caparoi showed the highest yield stability, although Hyperno was poor for screenings (increase of 6.2%) (Table 7). Overall, Caparoi showed the best balance of yield and grain size stability with late sowing, combined with high yield and grain size in the timely sowings, and low % screenings. These findings seem consistent with reports that Caparoi and Hyperno tend to give low and high % screenings, respectively, and that Saintly is able to yield relatively well in seasons when there is a high level of terminal stress.
Table 7. Performance ranking in August sowings relative to June sowings in tetraploid wheat genotypes, averaged across Leeton 2011 and Wagga Wagga 2012 irrigated field trials (lower values are more favourable in each case)1.
Genotype | Yield stability ranking | 1000-kw stability ranking | Increase in % screenings with later sowing | Test weight stability ranking | % grain protein gain ranking |
---|---|---|---|---|---|
Saintly | 1.0 | 9.0 | 2.0 | 7 | 8.5 |
Hypnerno | 2.0 | 9.0 | 6.2 | 5 | 8 |
Atil C2000 | 3.0 | 9.5 | 4.0 | 10.5 | 3 |
Caparoi | 4.0 | 3.0 | 1.1 | 6 | 8.5 |
WID 802 | 5.0 | 6.5 | 2.0 | 6 | 10.5 |
Yawa | 6.5 | 7.5 | 2.7 | 6 | 5.5 |
Kalka | 6.5 | 4.5 | 1.4 | 5 | 6.5 |
Tjilkuri | 8.0 | 4.0 | 3.8 | 13 | 3.5 |
Jandaroi | 9.0 | 6.0 | 0.5 | 3 | 10.5 |
Kronos | 10.0 | 3.5 | 0.7 | 3 | 9.5 |
Bellaroi | 11.0 | 10.5 | 3.4 | 11 | 2 |
Durati | 12.0 | 7.0 | 2.5 | 6 | 9 |
Tamaroi | 13.0 | 11.0 | 4.5 | 9.5 | 6 |
1All 12 trialled tetraploid varieties shown. Other details are the same as for Table 6.
Conclusions
Genotypes found to show heat tolerance in greenhouse/chamber tests, and/or stability of performance in late versus timely sowings in irrigated field trials, may carry heat tolerance that could protect against heat waves common to South Eastern Australian rainfed wheat cropping systems. We obtained evidence that the Sr2 rust resistance gene, present in many current Australian varieties, may worsen 1000-kw losses caused by heat events at grain filling.
However, this information should probably not be applied yet to influence grower variety choice. Notwithstanding a degree of wilting observed in plants in the heat treatment chamber or in the field on the hottest days, the well-watered conditions used in this study may have favoured expression of heat tolerance mechanisms based on transpirational cooling, which may be poorly expressed under regular rainfed conditions. Differences between greenhouse and regular field growing environments may affect expression of heat tolerance in unknown ways. Reductions in yield and grain size due to late sowing stem from the effects of accelerated development (due to higher average temperature in the ‘non-stressing range’ throughout growth), as well as from an increased incidence of heat waves at the reproductive stage; differences in genotype responses to all these factors potentially combined to influence the reported performance stability rankings.
It is therefore essential to test whether the heat tolerance effects reported here can be reproduced under rainfed, timely-sown field conditions. Our plan to achieve this in a cost effective manner is to identify chromosome regions affecting ‘tolerance’ using genetic analysis with the screening methods described herein, followed by construction of pairs of near-isogenic lines differing for the identified chromosome regions, and then to trial these near-isogenic lines to a high degree of replication under regular rainfed conditions. Towards this end, we have made near-isogenic lines for the described Drysdale x Waagan tolerance loci, and have used several of the tolerant genotypes identified here as parents in construction of new mapping populations.
Further work is therefore needed to realise a benefit of this project for heat tolerance breeding through the delivery of validated DNA markers. However, data from the two field trials could be directly relevant to irrigated wheat cultivation in NSW. For example, several CIMMYT hexaploid breeding lines and the cv. WaaganA showed potential for high yields under irrigation, and among these, HTWYT 001 and RT812 also showed good yield stability with delayed sowing, offering a good option if the grower is forced to sow late.
Meeting delivery standards for APH hexaploid wheat should be feasible under irrigation with timely sowing and good N management. However, low %HVK seems to pose a quality barrier for durum grown under irrigation with timely sowing. Meeting premium quality standards in hexaploid and durum wheat with (severely) delayed sowing also seems unlikely, at least with the varieties tested here, due to the effects of heat stress on test weight and screenings.
Acknowledgements
The research undertaken as part of this project is made possible by the significant contributions of growers through both trial cooperation and the support of the GRDC — the author would like to thank them for their continued support.
The authors would also like to thank the Australian Centre for Plant Functional Genomics (ACPFG) and NSW Department Primary Industries for additional support. The ACPFG was funded by the GRDC, ARC, the University of Adelaide and the South Australian Government. The Drysdale × Waagan work was partly funded by the NSW BioFirst initiative and GRDC project DAV00103.
References
Blumenthal C, Bekes F, Gras PW, Barlow EWR, Wrigley CW (1995) Identification of wheat genotypes tolerant to the effects of heat stress on grain quality. Cereal Chem 72:539–544
Brown GN (1997) The inheritance and expression of leaf chlorosis associated with gene Sr2 for adult plant resistance to wheat stem rust. Euphytica 95:67–71
Maphosa L, Collins N, Taylor J, Mather D (2014) Post-anthesis heat and a Gpc-B1 introgression have similar but non-additive effects in bread wheat. Funct Plant Biol 41:1002–1008
Saini H, Aspinall D (1982) Abnormal sporogenesis in wheat (Triticum aestivum L.) induced by short periods of high temperature. Ann Bot 49: 835–846
Shirdelmoghanloo H, Taylor JD, Lohraseb I, Rabie H, Brien C, Timmins A, Martin P, Mather DE, Emebiri L, Collins NC (2016b). A QTL on the short arm of wheat (Triticum aestivum L.) chromosome 3B affects the stability of grain weight in plants exposed to a brief heat shock early in grain filling. BMC Plant Biol 16:100
Shirdelmoghanloo H, Lohraseb I, Rabie HS, Brien C, Parent B and Collins NC (2016a). Heat susceptibility of grain filling in wheat (Triticum aestivum) linked with rapid chlorophyll loss during a 3-day heat treatment. Acta Physiol Plant 38:1–11
Sissons M, Ovenden B, Adorada D, Milgate A (2014) Durum wheat quality in high-input irrigation systems in south-eastern Australia. Crop & Pasture Science 65:411–422
Stone P, Nicolas M (1995) Effect of timing of heat stress during grain filling on two wheat varieties differing in heat tolerance. I. Grain growth. Funct Plant Biol 22:927–934
Stone P, Nicolas M (1996) Effect of timing of heat stress during grain filling on two wheat varieties differing in heat tolerance. II. Fractional protein accumulation. Aust J Plant Physiol 23:739–749
Sykes J (2012). Irrigated Wheat - Best Practice Guidelines In Cotton Farming Systems. Cotton Catchment Communities CRC.
Tashiro T, Wardlaw I (1990a) The effect of high temperature at different stages of ripening on grain set, grain weight and grain dimensions in the semi-dwarf wheat 'Banks'. Annals of Botany 65:51–61.
Tashiro T, Wardlaw I (1990b) The response to high temperature shock and humidity changes prior to and during the early stages of grain development in wheat. Funct Plant Biol 17:551–561
Contact details
Nick Collins
University of Adelaide, Waite Campus
PMB1, Glen Osmond SA, 5064
(08) 8313 7171; 0409 851 667
nick.collins@adelaide.edu.au
Was this page helpful?
YOUR FEEDBACK